Critical control points for proper mycotoxin management by Dr. Ir. Anouck Witters, Impextraco NV
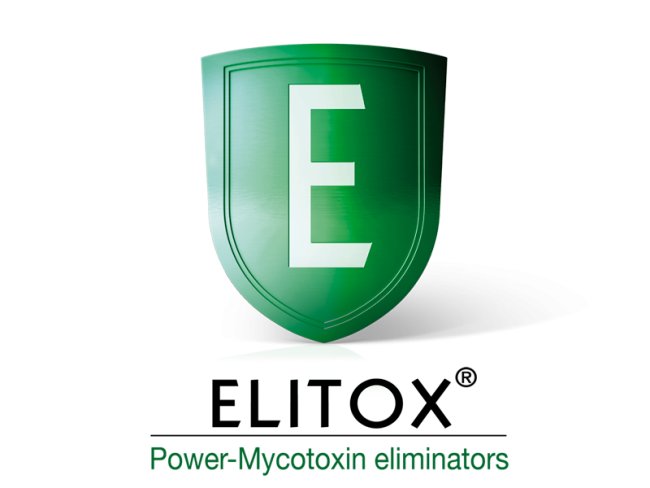
1. Introduction
Mycotoxins are naturally occurring toxic metabolites produced by fungi (moulds) in the field or during storage. Once the contaminated material is ingested by the animal, these metabolites cause mycotoxicosis. The toxicity of mycotoxins depends on the amounts ingested, duration of exposure, type of animal, their breed, age, sex, health status, but also on other parameters such as density of animals, diseases, temperature, etc… Diagnosis of mycotoxicosis is difficult as symptoms are usually non-specific.
2. Risk factors for mycotoxin contamination
The risk level for mycotoxins depends on climate conditions (temperature, humidity) since mold growth is favoured in hot and humid climates. Therefore, field as well as storage conditions need to be managed in order to minimize the risk for mycotoxin contamination. Nevertheless, equal field and storage conditions may face different mycotoxin problems according to the type of crops on the field. Including DDGS as a protein source in the feed, for example, implements an increased risk for mycotoxins (figure 1).
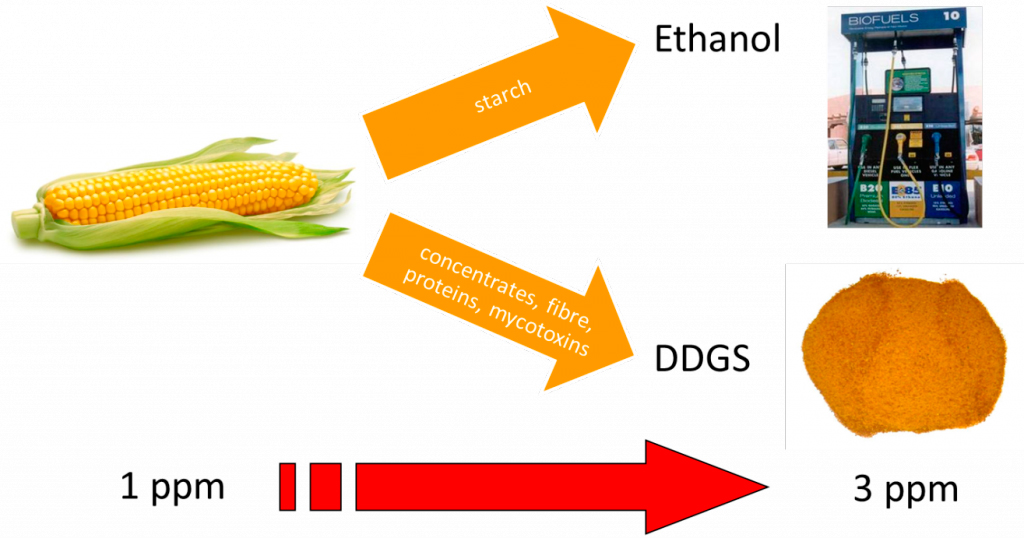
Fig. 1 The use of by-products and alternative feed ingredients implement increased risk on mycotoxin contamination. e.g. DDGS produced from corn with 1ppm mycotoxins is 3 times more contaminated than the source material.
In summary of these risk factors, mycotoxins seem to become an increasing problem on a global scale due to changes in global climatological conditions and increased worldwide trading in agricultural commodities. Moreover, by-products and alternative feed ingredients are being used more regularly, despite their high mycotoxin risk factor.
3. Detection of mycotoxins
The increased detection of mycotoxin contamination is not only due to an increased prevalence of mycotoxins, but also because of improved analytical methods to detect them. The first and most crucial step in mycotoxin analysis is sampling of raw materials or feed. Samples should be representative for the whole batch, which means that the sample should be taken at random but equally divided spots of the batch. Indeed, mycotoxin contamination can be concentrated to so-called ‘hot spots’, while other parts of the batch are not contaminated at all. On the other hand, the quantity of the sample should be proportional to the total batch weight.
Another detection-issue is the presence of so-called ‘masked’ mycotoxins. These toxins are bound to a more polar molecule, like glucose, resulting in conjugated mycotoxins. These conjugates have been shown to escape the routine mycotoxin detection methods; however, the toxic precursors will be released by hydrolysis during digestion. Literature reports occurrences of DON- and ZEA-glucoside up to respectively 12% of DON and 20% of ZEA levels (figure 2) (Schneweis et al., 2002; Berthiller et al., 2005). So, analyzing samples only for mycotoxins and not for their glucoside-conjugates, will probably underestimate the real risk for contamination.
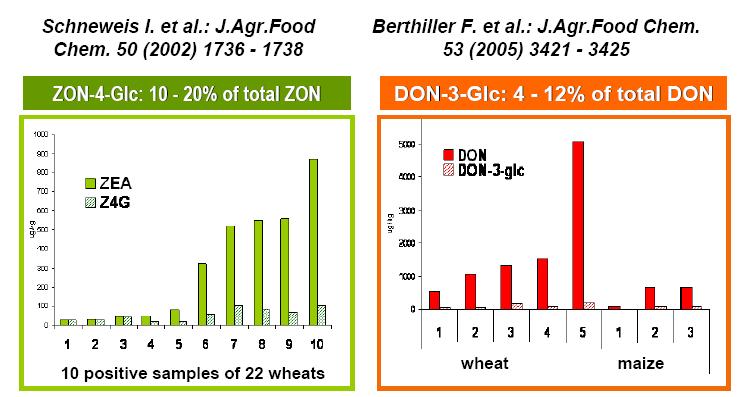
Fig. 2 Occurrence of mycotoxin glucoside-conjugates (ZON-4-Glc; DON-3-GLC) in maize and wheat samples according to 2 mini-surveys (Schneweis, I. et al., 2002; Berthiller, F. et al., 2005).
Besides the occurrence of masked and less-known mycotoxins; multi-mycotoxin contamination also means potential synergism between co-occuring toxins. It has been demonstrated that e.g. broiler body weight reduced 12% due to consuming 2.5ppm aflatoxin-contaminated feed; 0.2ppm ochratoxin-contaminated feed reduced the body weight by 14%; consuming feed contaminated with a combination of both mycotoxins resulted in 39% reduced body weights. Previous results clearly show the synergism between both toxins.
4. Prevention and control of mycotoxins
If favourable growth conditions for fungi are met, it is very difficult to avoid the production of mycotoxins. However, effective prevention strategies will certainly limit the incidence of mycotoxins. Prevention can be implemented before harvest with a good management of the crop, harvesting and storage. It must be noted, however, that prevention does not remove present mycotoxins.
Many methods, chemical as well as physical, have been tested to remove mycotoxins from commodities. The problem is that they are costly, usually generate high losses and may reduce the palatability and the nutritional value of the raw materials. In practice, mycotoxin binders are most commonly used, whether or not combined with the strategy of biotransformation.
4.1 Use of mycotoxin binders
Binding mycotoxins is a widespread approach to reduce the toxin’s bioavailability in the gastrointestinal tract. One of the first scientific studies on binding properties of clays showed that a specific type of phyllosilicate clays, hydrated sodium calcium aluminosilicates (HSCAS), have high affinity for aflatoxin B1 (Phillips et al., 1988). Indeed, the good stability of the aflatoxin-HSCAS complexes over a wide pH range (2-10) and up to 37°C supports the in vivo efficacy of such binders (Sarr et al., 1990). Further studies have demonstrated that HSCAS can be very helpful to prevent aflatoxicosis in different species such as chickens, turkeys, goats, cows, pigs, or lambs. However, the efficacy of HSCAS seems to differ according to the type of mycotoxins: HSCAS are effective against aflatoxin B1 and ochratoxin A, while they appear totally ineffective to tackle trichothecenes (e.g. T-2 toxin, deoxynivalenol).
Although activated carbon has proven to be effective on binding mycotoxins in vitro, e.g. fumonisin B1 (Solfrizzo et al., 2001) or ochratoxin A, it did not show clear positive effects in vivo. Probably, this could be due to the fact that activated carbon can indiscriminately bind other dietary components, such as vitamins, minerals and drugs.
Stanley et al. (1993) reported Saccharomyces cerevisiae being helpful in the case of aflatoxin contamination; they concluded that the yeast cell wall binds mycotoxins. The effects of yeast cell walls against ochratoxin were studied by Santin et al. (2003) in broilers. Their results indicate that zootechnical parameters, impaired due to ochratoxins, were not improved by application of yeast cell walls. Yiannikouris et al. (2004) investigated the interaction of yeast cell walls with zearalenone in vitro. They concluded that the chemical interactions are better described as adsorption rather than binding.
Based on literature, the main limitations of “mycotoxin binders” can be summarized as following:
- Their efficacy is limited to a few mycotoxins.
Generally speaking, binders are effective against so-called polar mycotoxins, such as aflatoxins. Indeed, these mycotoxins have a chemical structure which allows efficient binding. In the case of other mycotoxins, such as trichothecenes, binding efficacy is generally very poor, if not zero.
- Their in vitro efficacy does not guarantee their performance in vivo.
In vitro tests do not always represent what happens in the digestive tract as they are performed under specific and rather simple conditions. Not taking into account variable physiological parameters (pH variation, interaction with feed or enzymatic secretions) increases the risk to draw false conclusions. Indeed, mycotoxins may be released from the binder in the intestinal environment, as it is a reversible process.
- Some of them are not specific to mycotoxins.
Some binders interact with other dietary components, such as vitamins, minerals and drugs. This will limit the efficacy against the mycotoxin(s) and also affect the performance of the animals.
4.2 Biotransformation of mycotoxins
Due to the above listed drawbacks of mycotoxin binders, alternative strategies are recommended. By means of live micro-organisms or enzymatic preparations, mycotoxins are biotranfsformed into non-toxic metaboliltes.
An Agrobacterium-Rhizobium was reported to be able to transform deoxynivalenol into a less toxic compound, 3-keto-deoxynivalenol (Shima et al., 1997). This biotransformation was suggested to be caused by an extracellular enzyme excreted by the organism. A similar transformation of trichothecenes was observed by Völkl et al. (2004).
Zearalenone, which interferes with oestrogen receptors, can be converted into a far less oestrogenic-like product, 1-(3,5-dihydroxyphenyl)-10’-hydroxy-1’-undecen-6’-one (Takahashi-Ando et al., 2002). The enzyme responsible for the detoxification appears to be a hydrolase that cleaves the lactone ring.
The application of such enzymatic transformations in the feed sector offers new opportunities. Indeed, compared to binders, enzymes can have a higher specificity and perform a non-reversible reaction. Moreover, the enzyme activity is not limited to polar mycotoxins only.
5. Evaluating efficacy of mycotoxin controlling products
To reveal the real binding or inactivation of mycotoxins, simple measurements in the feed (in vitro) are not sufficient since they do not reflect actual conditions inside the animal and the possible interactions with binding or enzymatic reaction.
Nevertheless, analyzing the toxin inactivating effect in live animals (in vivo) is very complex. Most of the studies evaluate animal performance; however, this parameter is influenced by many other factors difficult to control. Some studies measure the serum mycotoxin levels. However, this is not possible for all types of mycotoxins and most toxins are rapidly metabolised or stored in the animal. Consequently, the serum levels are not always representative for the ingested amount of mycotoxins. Moreover, individual serum mycotoxin levels also differ according to genetics, bodyweight, water intake etc. of the animal.
A perfect in vitro model of the animal eliminates individual variation and controls all other factors. At HAS Den Bosch (High School of Agriculture, the Netherlands) a small intestinal model was developed that simulates anaerobic environment, constant (body) temperature, several subsequent environments at different pH, retention times, addition of bile, pepsin and gut enzymes and the moisture:feed (digestive bolus) ratio (figure 3). So, this model allows studying interactions between feed, mycotoxins and mycotoxin-deactivating substances in “real” conditions. This offers a clear advantage compared to the classical in vitro tests where only pH is controlled and other parameters are not taken into account.
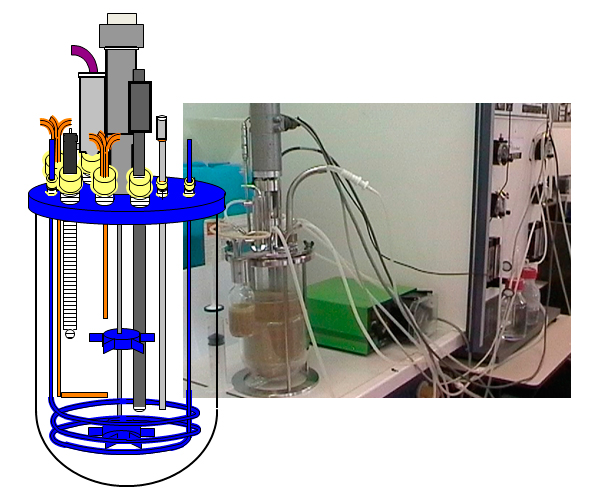
Fig. 3 Gut simulator model developed by HAS Den Bosch research group (the Netherlands). This model was used by Impextraco (Belgium) to develop their feed additive against mycotoxins, Elitox®.
In the development of a solution to counteract the effects of mycotoxins in feed, Impextraco (Belgium) screened many products, including binders and enzymes, by means of this gut simulator model. The best toxin binder that emerged from this screening was combined with enzymes. In addition, a biopolymer was selected for its mycotoxin binding properties. Moreover, the biopolymer has been proven to be antibacterial and antifungal. The included natural extracts and vitamins act against the symptoms associated with mycotoxin contamination. In conclusion, Impextraco’s product (Elitox®) unites in one single product different strategies to counteract a wide range of mycotoxins.
6. Conclusion
Mycotoxins are harmful to animals and can greatly affect their performance and productivity. Because of the wide range of mycotoxins, with different chemical structures, a single approach cannot efficiently solve the problem.
Prevention is a key control point but cannot guarantee the absence of mycotoxins. Contaminated commodities require the use of different elimination strategies. A product combining mycotoxin binders with toxin-degrading enzymes and a biopolymer seems to be the required standard for proper mycotoxin management.
References are available upon request.